The Geiger-Müller (GM) Counter: A Milestone in Radiation Detection
- bpsinghamu
- Mar 27
- 5 min read
In the dimly lit laboratory of the early 20th century, a young physicist named Hans Geiger meticulously worked on a device that could detect the invisible yet powerful presence of radiation. It was 1908, and the mysteries of atomic particles were just beginning to unfold. Geiger's early detector was rudimentary, capable of detecting alpha particles, but it lacked efficiency and sensitivity. It wasn’t until 1928, when he collaborated with Walther Müller, that the Geiger-Müller tube was perfected—a revolutionary instrument that would change the course of radiation detection forever.
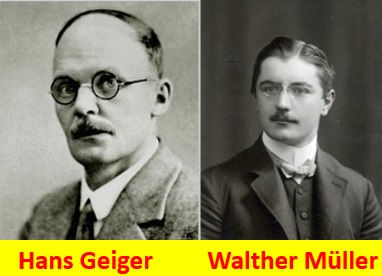
Hans Geiger, born in 1882 in Neustadt an der Weinstraße, Germany, was a pioneering experimental physicist best known for his contributions to radiation detection and nuclear physics. His father, Wilhelm Ludwig Geiger, was a professor of linguistics. Hans studied physics and mathematics at the University of Erlangen, earning his doctorate in 1906. He later joined Ernest Rutherford at the University of Manchester, where he helped develop early methods for detecting alpha particles. His expertise in radiation detection led him to conceive the first Geiger counter.
Walther Müller, born in 1905 in Hanover, Germany, was Geiger’s doctoral student. His father was a school teacher, fostering an early interest in academics. Müller studied physics at the University of Kiel, where he collaborated with Geiger to refine the detector, significantly improving its sensitivity and efficiency. His modifications allowed the device to detect not only alpha particles but also beta and gamma radiation, making it an indispensable tool in scientific research and industry. The collaboration between Geiger and Müller resulted in one of the most widely used radiation detectors in history-popularly called GM Counter.
The GM tube, at its core, was a simple yet ingenious device. A cylindrical chamber filled with gas (preferable Argon and Methane) housed a fine (thin) central wire acting as an anode, while the outer shell (generally made of Copper) served as the cathode. See Figure below. A high voltage applied across these electrodes created an invisible electric field (radial electric field in case of cylindrical geometry), waiting for an unsuspecting nuclear radiation to arrive. When ionizing radiation passed through (shown by arrow) it struck the gas molecules, liberating electrons and setting off a chain reaction of ionization known as the Townsend avalanche.
It may be mentioned that on an average about 32 eV of energy is requited to create one electron-ion pair. A tiny but distinct electrical pulse was generated with each interaction. The electrical pulse could be counted meticulously by an external circuit—each click a testament to the presence of radiation.
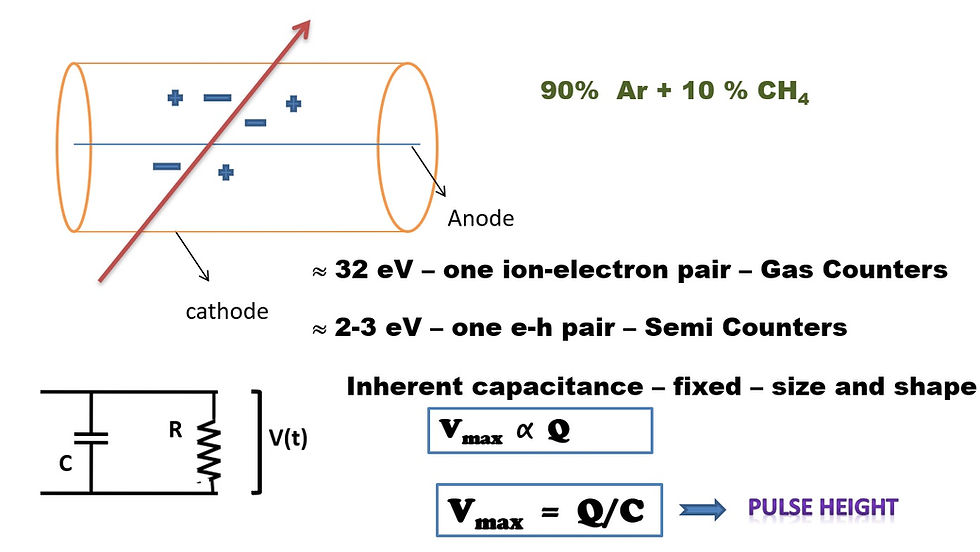
A Geiger-Müller counter is like a radiation detector that listens for invisible particles. Inside its tube, there’s gas and a wire with high voltage. When radiation enters, it knocks electrons off the gas atoms, creating a chain reaction. This reaction produces a small electrical pulse, which gets counted and converted into the familiar 'click' sound. The more radiation, the more clicks! This simple yet effective mechanism helps scientists and safety professionals detect radiation levels.

As word spread of this remarkable invention, laboratories and industries alike embraced the GM counter. It became an essential tool for physicists exploring the depths of nuclear reactions, for engineers ensuring radiation safety in power plants, and for doctors safeguarding patients in medical imaging and therapy. Environmental scientists carried handheld GM counters into fields and forests, monitoring radioactive contamination with precision. Its versatility was unmatched, capable of detecting alpha, beta, and gamma radiation, depending on its construction and features.

In the decades that followed, the GM tube continued to evolve. Its applications expanded beyond research and industry to personal safety. Miniaturization allowed workers in nuclear facilities to carry pocket-sized dosimeters, alerting them to unseen dangers. The rise of digital technology transformed radiation monitoring, enabling real-time data logging and even smartphone integration. Despite competition from scintillation and semiconductor detectors, which could distinguish radiation types and energies, the GM tube remained a trusted companion for countless professionals due to its affordability, simplicity, and reliability.
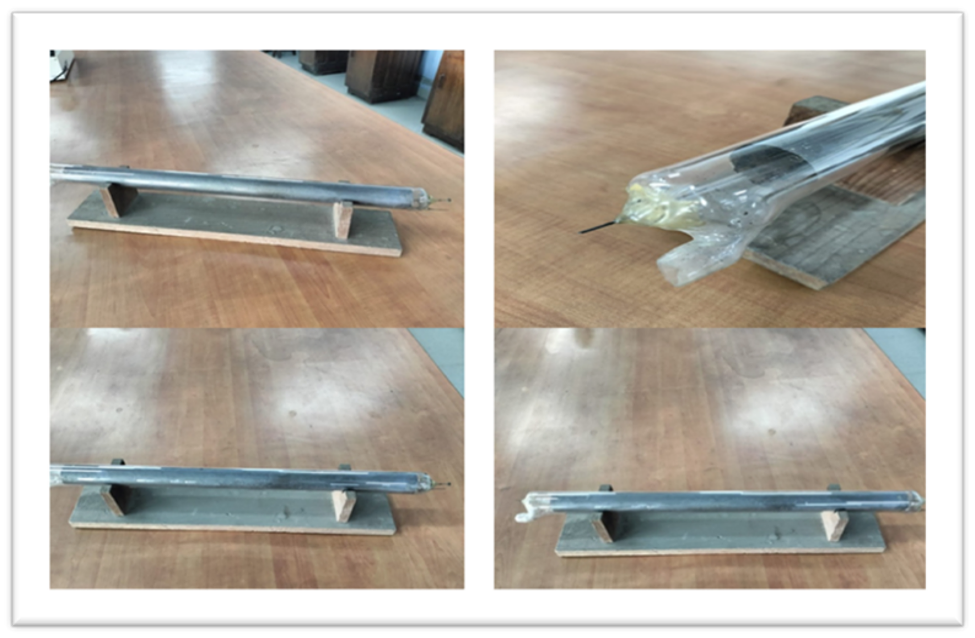
GM Counters preserved in the Heritage Science Museum
Comments